2009 Drosophila Image Award
Winner
Two-photon microscopy and analysis of Histone2A-GFP expressing embryos captures key events in gastrulation
Posterior and dorsal views of mesoderm cells as they spread to form a monolayer during gastrulation. Cells are imaged live with a nuclear marker (H2A-gfp). The imaging data is then imported into data-processing software to segment and follow each nuclei over time (colored lines, blue=early time points, yellow=late time points). The orange spheres represent mesoderm nuclei as they undergo gastrulation. The ectoderm, which is the substratum for mesoderm cell migration, is shown for reference (white). The total displacement is displayed for both the mesoderm (orange arrows) and ectoderm (white arrows).
McMahon, Supatto, Fraser, Stathopoulos Science 322, 1546 (2008)
Runner-Up
Dynamic cell matching during dorsal closure in live Drosophila embryos
Dorsal closure is a morphogenetic movement occurring late in Drosophila embryogenesis, in which two epithelial sheets fuse in order to seal a hole in the epidermis. The epidermis is segmentally patterned and it is essential that the epithelia fuse accurately during dorsal closure, as this patterning must be maintained across the fusion seam. To study how this accuracy was achieved, we live imaged dorsal closure in embryos expressing GFP-Moesin in anterior (A) compartment cells and RFP-Moesin in posterior (P) compartment cells. Accuracy is achieved by a cell matching mechanism, in which filopodia on the leading edge epidermal cells recognize and specifically fuse with cells in equivalent positions in the opposing epithelium. As the filopodia can reach several cell widths, this allows misalignments to be corrected.
Tom Millard & Paul Martin (2008) Dynamic analysis of filopodial interactions during the zippering phase of Drosophila dorsal closure. Development 135 621-626.
Runner-Up
Molecular zip codes for odor receptor gene choice in Drosophila
Scanning electron micrograph of a Drosophila head overlaid with expression patterns of four odor receptor genes in the olfactory neurons of the antenna (blue, yellow, and magenta) and the maxillary palp (green). Sequences represent cis elements conserved upstream of odor receptor genes across 12 Drosophila species that specify expression of odor receptor genes in specific neuronal classes.
Credit: Design by Anandasankar Ray and Woodstock Tom; SEM by Jennifer Perry.
Anandasankar Ray, Wynand van der Goes van Naters and John R. Carlson.
A Regulatory Code for Neuron-Specific Odor Receptor Expression.
PLoS Biology (2008). 6(5):doi:10.1371/journal.pbio.0060125å
Finalist
Dynamic cell matching during dorsal closure in live Drosophila embryos
Dorsal closure is a morphogenetic movement occurring late in Drosophila embryogenesis, in which two epithelial sheets fuse in order to seal a hole in the epidermis. The epidermis is segmentally patterned and it is essential that the epithelia fuse accurately during dorsal closure, as this patterning must be maintained across the fusion seam. To study how this accuracy was achieved, we live imaged dorsal closure in embryos expressing GFP-Moesin in anterior (A) compartment cells and RFP-Moesin in posterior (P) compartment cells. Accuracy is achieved by a cell matching mechanism, in which filopodia on the leading edge epidermal cells recognize and specifically fuse with cells in equivalent positions in the opposing epithelium. As the filopodia can reach several cell widths, this allows misalignments to be corrected.
Tom Millard & Paul Martin (2008) Dynamic analysis of filopodial interactions during the zippering phase of Drosophila dorsal closure. Development 135 621-626.
Finalist
Organization and dynamics of adhesion molecules at cell contacts
How does cell-cell adhesion ensure both tissue cohesion and remodeling? We have studied the organization and dynamics of E-cadherin molecules in living embryos and shown that the actin cytoskeleton (magenta) controls independently the stability of E-cadherin (green) and its mobility at cell contacts.
An E-cadherin::GFP fusion forms very stable puncta (circled at top) as shown by Fluorescence Recovery After Photobleaching (FRAP) analysis (left panel, arrow). Stable patches of actin filaments stabilize E-cadherin in puncta.
Most puncta are immobilized at cell contacts (middle panel) by a second population of actin filaments with high turnover, organized as a cortical contractile network.
Laser nano-sectioning of this cortical network (X) shows that E-cadherin puncta immobility is mediated by tethering of E-cadherin to this network via -catenin.
We propose a model whereby E-cadherin clusters in puncta form adhesion units with distinct regulation of stability and mobility. This provides a simple framework to explain tissue cohesion and remodeling.
Cavey M, Rauzi M, Lenne PF, Lecuit T. A two-tiered mechanism for stabilization and immobilization of E-cadherin. Nature. 2008 Jun 5; 453(7196):751-6.
Finalist
Drosophila egg-laying site selection as a system to study simple decision-making processes
The ability to select a better option from multiple acceptable ones is important for animals to optimize their resources. We explored the possibility of using egg-laying site selection in Drosophila melanogaster as a system to study simple decision-making processes. We found that Drosophila females can either accept or reject a sucrose-containing medium for egg-laying depending on context: in the absence of other choices,Drosophila females accept the sucrose medium as a egg-laying site; they will reject the sucrose medium, however (green substrate), if the sucrose medium is paired with a plain medium or a lobeline-containing medium (red substrate). This finding is consistent with the idea that selection of egg-laying site involves a simple decision-making process.
Yang, C.H., Belawat, P., Hafen, E., Jan, L.Y., and Jan, Y.N. (2008). Drosophila egg-laying site selection as a system to study simple decision-making processes.Science 319, 1679-1683
Finalist
Pulsed actomyosin contraction drives apical constriction
Apical constriction promotes the bending and invagination of epithelial tissues. Apical constriction is conventionally thought to be driven by the continuous contraction of an actomyosin purse-string. However, this movie of myosin (green) and cell outlines (red) shows that ventral furrow cells constrict incrementally via pulses of myosin contraction. This ‘ratchet’ mechanism for apical constriction could play a role in coordinating the behavior of groups of cells.
Martin, A. C., Kaschube, M. & Wieschaus, E. F. Pulsed contractions of an actin-myosin network drive apical constriction. Nature advance online publication, doi:10.1038/nature07522 (23 November 2008).
Finalist
Transit-amplifying neuroblast lineages in the larval brain
Throughout embryonic and larval development, neural precursor cells called neuroblasts divide in a self-renewing manner and produce large numbers of small, differentiating daughter cells. These daughter cells eventually give rise to the neurons and glia of the central nervous system. It was previously thought that all neuroblast daughters are ganglion mother cells (GMCs) — cells that divide terminally to produce differentiated neurons or glia. We found that a distinct subpopulation of larval neuroblasts do not produce ganglion mother cells, but instead generate small, secondary neuroblasts. The secondary neuroblast acts as an intermediate precursor, dividing several times to give rise to multiple GMCs. Adding this transit-amplification step to the neuroblast lineage allows production of GMCs and neurons at a faster rate.
In these complementary images of a single larval brain lobe, primary neuroblasts appear as large circles outlined by phalloidin staining (left, green; right, blue). Primary neuroblasts of the classical lineages express the neural precursor marker Asense (red). An asensereporter (green, right, asense-Gal4 >> CD8-GFP) is also expressed in the classical neuroblast and many of its progeny. By contrast, primary neuroblasts of the transit-amplifying lineages do not express Asense or the asense reporter. The asense reporter is not detectable in small secondary neuroblasts (right), even though they express Asense protein.
Sarah K. Bowman, Vivien Rolland, Joerg Betschinger, Kaolin A. Kinsey, Gregory Emery, and Juergen A. Knoblich (2008). The Tumor Suppressors Brat and Numb Regulate Transit-Amplifying Neuroblast Lineages in Drosophila. Developmental Cell 14: 535-546.
Finalist
A Double S Shape Scaffold Underlies the Extraordinary Binding Specificity of Dscam Isoforms
Drosophila Dscam encodes 38,016 distinct axon guidance receptors. This diversity is essential for wiring the fly brain. Dscam isoforms exhibit striking binding specificity – each binds homophilically to itself but not heterophilically to other isoforms. To assess how this binding specificity is achieved we solved the structure of the Dscam homophilic binding complex which reveals the molecular basis for Dscam binding specificity. Dscam isoforms contain three variable domains (red, blue, and green) which each bind in a two-fold symmetric fashion to an identical domain in the opposing molecule. To accommodate these three interfaces, Dscam proteins adopt a unique S shape giving the homophilic binding complex an elegant double S shape.
Michael R. Sawaya, Woj M. Wojtowicz, Ingemar Andre, Bin Qian, Wei Wu, David Baker, David Eisenberg and S. Lawrence Zipursky. (2008) A Double S Shape Provides the Structural Basis for the Extraordinary Binding Specificity of Dscam Isoforms. Cell 134:1007-1018.
Finalist
The importance of keeping a stiff actin cell cortex at mitosis
The Mitotic spindle protrudes from the cell cortex and the chromosomes are misaligned when Drosophila SR+ cells treated with dsRNA for Moesin, the actin-membrane crosslinker protein, are grown in suspension (left). This defect in mitosis demonstrates the necessity to establish a stiff cortex for the proper mitotic spindle organization and chromosome segregation. These errors are the principal cause of aneuploidy which have been implicated in several aberrant diseases including cancer. The phenotype in Moesin knock down cells can be rescued by increasing cortical tension from the outside by addition of tetravalent lectin Concavalin A to the cell suspension (right). This shows that restitution of cortical tension restores the normal spindle morphology in the cells with low levels of Moesin. F-actin (red), microtubules (green), and phospho-Histone 3 (blue). Scale bar 10 mm.
Patricia Kunda, Andrew E. Pelling, Tao Liu and Buzz Baum. (2008) Moesin controls cortical rigidity, cell rounding, and spindle morphogenesis during mitosis.Curr Biol. 18, 91-101.
Finalist
Conservation of Gene Content in the Chromosomal Arms among Drosophila species
In the 1940s, Sturtevant, Tan, Novitski, and Muller established that the gene content of the chromosomal arms of species in the genus Drosophila was conserved. The annotation of the 12 genomes confirms that chromosomal arms are conserved among Drosophila species, but that gene order is extensively shuffled. This image represents each gene as a colored line and each chromosomal arm is indicated in a different color. The homologous genes are connected by lines that show the extensive rearrangement of gene order among Drosophila species. Arbitrary blocks of genes in D. grimshawi were colored to allow one to visualize the degree of rearrangement among species.
Bhutkar, A. J., S.W. Schaeffer, S. Russo, M. Xu, T. F. Smith, W. J. Gelbart (2008). Chromosomal rearrangement inferred from comparisons of twelve Drosophila genomes. Genetics 179: 1657-1680.
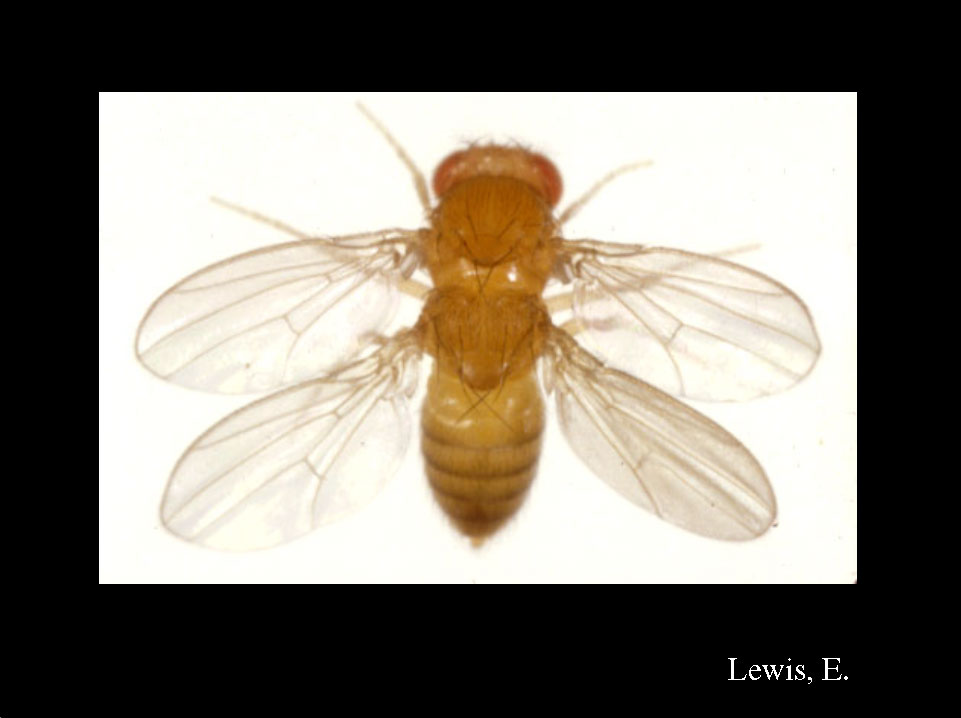