2020 Drosophila Image Award
Winner – Video
Microcomputed tomography (μ-CT) as a whole fly phenotyping tool
An adult female is shown in 3D and digitally sliced along the XZ and YZ axes to reveal all intact internal structures. Each organ is then sequentially highlighted in color, beginning with anterior structures. The non-destructive nature of the technique permits high resolution imaging at any developmental stage, preserving the spatial architecture of all organs. This makes μ-CT a powerful tool for unbiased characterization of developmental defects and a deeper understanding of inter-organ relationships.
Video Credit: Todd A. Schoborg
Schoborg TA, Smith SL, Smith LN, Morris HD, Rusan NM
Micro-computed tomography as a platform for exploring Drosophila development.
Development. 2019 Dec 11;146(23)
Winner – Still Image
A depth color-coded confocal microscopy image of fruit fly reproductive organs during coitus
The image shows the cuticle of Drosophila genitals in coitus. Genital coupling through interlocking of complementary surfaces is a vital aspect of successful copulation. The genitals (female’s on top) were imaged at 400x magnification with a laser scanning confocal microscope and presented as depth color-coded maximum intensity projection after application of ‘find edges’ filter
Image Credit: Igor Siwanowicz (Drosophila Connectomics Group, Cambridge)
Shao L, Chung P, Wong A, Siwanowicz I, Kent CF, Long X, Heberlein U
A Neural Circuit Encoding the Experience of Copulation in Female Drosophila.
Neuron. 2019 Jun 5;102(5):1025-1036
Runner Up
Class I Proprioceptive Neurons in a Freely Moving Larva
The 2nd instar Drosophila larva was positioned and freely moving in a water filled channel on a cover glass. The class I proprioceptive neurons are co-labelled with GCaMP6f and tdTomato. Volumetric imaging was achieved with the SCAPE microscope. The entire 3D field of view (930um x 420um x 168um , X-Y-Z) from the ventral side of the larva was imaged at 10 volumes per second for dual color channels simultaneously, in order to capture the rapid movement and the neuronal activity of the crawling larva. Individual proprioceptive neurons was tracked and the calcium activity was plotted with the rhythmic segment contraction during forward crawling.
Video Credit: Wenze Li
Vaadia RD, Li W, Voleti V, Singhania A, Hillman EMC, Grueber WB
Characterization of Proprioceptive System Dynamics in Behaving Drosophila Larvae Using High-Speed Volumetric Microscopy.
Curr Biol. 2019 Mar 18;29(6):935-944
Runner Up
Branches of Tracheal Terminal Cells Invading Myotubes
In the developing flight muscles, branches of tracheal terminal cells invade the myotubes and ramify within them to provide oxygen supply. Stochastic multicolor labeling revealed that individual tracheal cells occupy largely non-overlapping territories within the myotube, suggesting that invading tracheal cells minimize overlaps, possibly mediated by contact-dependent repulsion.
Image Credit: Julia Sauerwald and Stefan Luschnig
Sauerwald J, Backer W, Matzat T, Schnorrer F, Luschnig S
Matrix metalloproteinase 1 modulates invasive behavior of tracheal branches during entry into Drosophila flight muscles.
Elife. 2019 Oct 2;8
Honorable Mention
Branches of Tracheal Terminal Cells Invading Myotubes
In the developing flight muscles, tracheal branches invade the myotubes and ramify within them to provide oxygen supply. 3D animation of a z-stack of a single myotube stained for F-actin (magenta), LaminDm0 (cyan; all nuclei) and DSRF (yellow; tracheal terminal cell nuclei. Tracheal branches (green) were visualized by their autofluorescence.
Video Credit: Sauerwald J, Backer W, Matzat T, Schnorrer F, Luschnig S
Sauerwald J, Backer W, Matzat T, Schnorrer F, Luschnig S
Matrix metalloproteinase 1 modulates invasive behavior of tracheal branches during entry into Drosophila flight muscles.
Elife. 2019 Oct 2;8
Honorable Mention
DeepFly3D measures Drosophila 3D limb movements
One fundamental requirement to understand how neural dynamics govern behavior is to precisely measure behavior in 3-dimensions (3D). Recently, deep networks and computer vision approaches have been successfully applied to measure 3D limb movements. For example, this figure shows how our software, DeepFly3D, solves this problem for Drosophila. DeepFly3D takes (A) raw images from each of seven cameras as inputs to a deep network, (B) outputs joint location probability, (C) generates 2D pose estimates after applying pictorial structures and multi-view algorithms, (D) derives 3D pose estimates derived by combining 2D pose estimates from multiple camera views, and (E) finally outputs 3D pose for the six legs.
Image Credit: Semih Günel and Pavan Ramdya
Günel S, Rhodin H, Morales D, Campagnolo J, Ramdya P, Fua P
DeepFly3D, a deep learning-based approach for 3D limb and appendage tracking in tethered, adult Drosophila.
Elife. 2019 Oct 4;8
Honorable Mention
Analysis of a pupal lethal mutation, caused by tissue specific knockdown of spc105r
3D μ-CT rendering of a wildtype and spc105r pupa at P11. The brain (blue) and ventral nerve cord (red) are highlighted, as is the position of the head (WT) and head remnant (yellow, spc105r mutant). Imaging revealed the presence of a partially developed brain located in the thorax of the spc105r mutant, despite the lack of other head structures. This demonstrates the usefulness of μ-CT to identify new or overlooked phenotypes.
Video Credit: Todd A. Schoborg
Schoborg TA, Smith SL, Smith LN, Morris HD, Rusan NM
Micro-computed tomography as a platform for exploring Drosophila development.
Development. 2019 Dec 11;146(23)
Honorable Mention
Dendritic arbor morphology of a yDm8 amacrine neuron contacting R7 photoreceptors visualized with expansion microscopy
This image is a high-resolution view of contacts made between ‘yellow’ Dm8 dendrites (yDm8, cyan) and R7 photoreceptor axon terminals (magenta) in the Drosophila optic lobe. A single yDm8 neuron labeled with GFP and R7 terminals labeled with photoreceptor-specific marker Chaoptin was examined with expansion microscopy and surface rendered with Imaris software. Drosophila R7 UV photoreceptors (PRs) are divided into yellow (y) and pale (p) subtypes. yR7 PRs express Dpr11 cell surface protein and are presynaptic to Dm8 amacrine neurons (yDm8) that express Dpr11’s binding partner DIP-, while pR7 PRs synapse onto DIP--negative pDm8. Dpr11 and DIP- expression patterns define ‘yellow’ and ‘pale’ color vision circuits which take photoreceptor signals and transform them to color percepts for guiding their behavior in the natural world. Our study shows how the yellow circuit is built using Dpr11 and DIP- as molecular determinants.
Image Credit: Kaushiki Menon
Menon KP, Kulkarni V, Takemura SY, Anaya M, Zinn K
Interactions between Dpr11 and DIP-γ control selection of amacrine neurons in Drosophila color vision circuits.
Elife. 2019 Nov 6;8
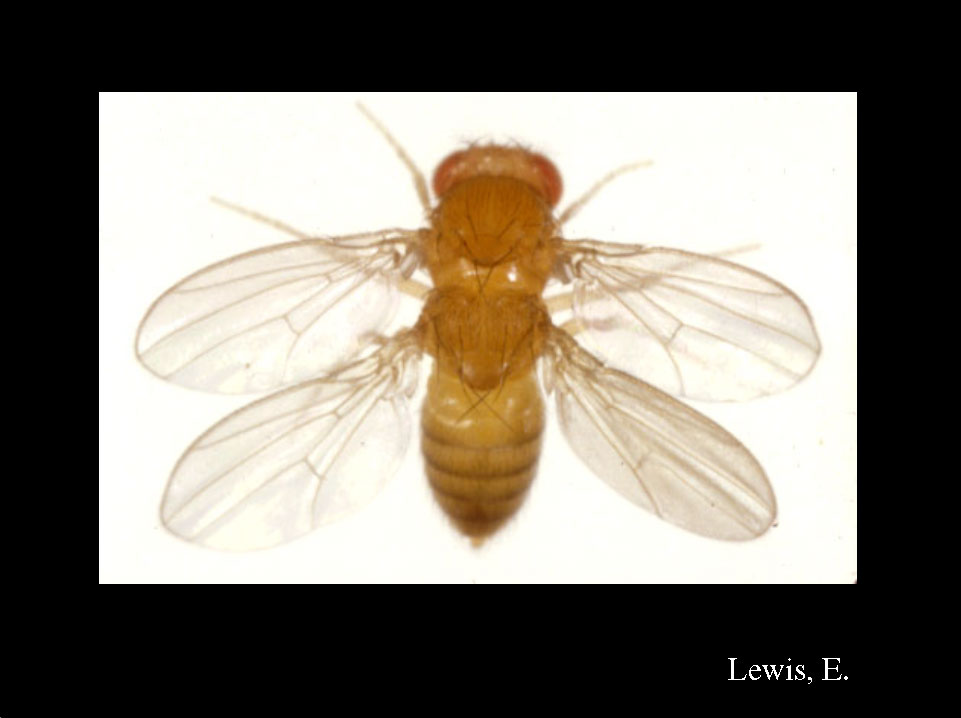