2015 Drosophila Image Award
Winner – Video
Systems Analysis of the Dynamic Inflammatory Response to Tissue Damage
Following tissue damage, a complex repair process is initiated to restore the integrity of the injured tissue. Re-epithelialisation is accompanied by a rapid inflammatory response, whereby cells of the innate immune system (here, Drosophila macrophages called ‘hemocytes’) are rapidly recruited to the damaged area. Although we can live-image this process with high spatial and temporal resolution in vivo, the precise identity and properties of the immune damage attractants remain unclear.
Here, we employ an alternative computational approach to extract detailed information about the wound attractant signal from the in vivo dynamic behaviour of the responding immune cells. Using this novel approach, we have uncovered new insight into the spatio-temporal properties of the wound attractant, revealing precisely how the damage signal spreads out from the injury site in space and time, and inferring parameters such as the diffusion coefficient, source, and duration of active signal production. Our study highlights the valuable insight that can be extracted from in vivo imaging data if more sophisticated analysis tools are employed, that would otherwise remain experimentally inaccessible.
Weavers H, Liepe J, Sim A, Wood W, Martin P & Stumpf M. (2016)
Systems Analysis of the Dynamic Inflammatory Response to Tissue Damage Reveals Spatiotemporal Properties of the Wound Attractant Gradient
Current Biology 26, 1975-1989 (2016)
doi:10.1016/j.cub.2016.06.012
Winner – Still Image
Nutritional regulation of intestinal trachea
This image shows the nutritional plasticity of a terminal tracheal cell (visualised in green using DSRF>mCD8GFP) populating the larval hindgut (highlighted in blue with an actin staining). In well fed larvae, the enteric tracheal terminal cells are profusely branched (top panel). A mild dietary restriction that does not affect growth rate or the size of other organs results in preferentially reduced branching of these enteric tracheal cells (bottom panel), but not other tracheal subsets.
Our study further showed that tracheal branching is controlled by nutrient responsive-neurons through their release of insulin-like and Pigment-dispersing factor (Pdf) neuropeptides. It also demonstrated that the plasticity of enteric trachea plays a key role in driving adaptations to malnutrition. Together, our findings uncovered a new mechanism by which nutritional cues modulate neuronal activity to give rise to organ-specific, long-lasting changes in tracheal architecture.
Linneweber GA, Jacobson J, Busch KE, Hudry B, Christov CP, Dormann D, Yuan M, Otani T, Knust E, de Bono M, et al.
(2014). ”Neuronal Control of Metabolism through Nutrient-Dependent Modulation of Tracheal Branching.” Cell 156, 69-83.
The Mushroom Body Output Neurons
Mushroom body is a center for associative learning in insect brains. The movie shows comprehensive set of mushroom body output neurons (MBONs) of adult Drosophila in the standard brain after image registration. Original confocal images were obtained by using split-GAL4 drivers for each MBON cell type. These MBONs collectively tile entire mushroom body lobes with minimal overlap. Identification of a full set of MBONs and generation of split-GAL4 facilitates understanding of memory circuit in fly brains.
The neuronal architecture of the mushroom body provides a logic for associative learning.
Yoshinori Aso, Daisuke Hattori, Yang Yu, Rebecca M Johnston, Nirmala A Iyer, Teri-TB Ngo, Heather Dionne, LF Abbott, Richard Axel, Hiromu Tanimoto, Gerald M Rubin
Nutritional regulation of intestinal trachea
This image shows the nutritional plasticity of a terminal tracheal cell (visualised in green using DSRF>mCD8GFP) populating the larval hindgut (highlighted in blue with an actin staining). In well fed larvae, the enteric tracheal terminal cells are profusely branched (top panel). A mild dietary restriction that does not affect growth rate or the size of other organs results in preferentially reduced branching of these enteric tracheal cells (bottom panel), but not other tracheal subsets.
Our study further showed that tracheal branching is controlled by nutrient responsive-neurons through their release of insulin-like and Pigment-dispersing factor (Pdf) neuropeptides. It also demonstrated that the plasticity of enteric trachea plays a key role in driving adaptations to malnutrition. Together, our findings uncovered a new mechanism by which nutritional cues modulate neuronal activity to give rise to organ-specific, long-lasting changes in tracheal architecture.
Linneweber GA, Jacobson J, Busch KE, Hudry B, Christov CP, Dormann D, Yuan M, Otani T, Knust E, de Bono M, et al.
(2014). ”Neuronal Control of Metabolism through Nutrient-Dependent Modulation of Tracheal Branching.” Cell 156, 69-83.
Ack kinase localizes to CTP synthase filaments in Drosophila ovarian germ cells
The non-receptor tyrosine kinase Ack (activated cdc42-associated kinase) localizes to filaments composed of the nucleotide biosynthetic enzyme CTP synthase in nurse cells of Drosophila egg chambers. Female flies deficient in Ack catalytic activity have morphologically abnormal CTP synthase filaments, reduced RNA levels, membrane phospholipid defects, and decreased fertility, phenotypes associated with decreased CTP synthesis. These findings suggest that Ack regulates CTP synthase enzymatic activity during Drosophila oogenesis. Shown is a 3-D confocal projection of egg chambers expressing GFP-CTP synthase (green) and stained with an antibody to detect Drosophila Ack. Co-localization in indicated in yellow and nuclei are shown in blue (stained with Draq5).
Kanca et al. , Raeppli: a whole-tissue labeling tool for live imaging of Drosophila development, Development (2014) 141, 472-480 doi:10.1242/dev.102913
Through the pupal case imaging of wing opening
The unfolding of the wing in while pupal stages is imaged through the pupal case. Each clonal territory is genetically marked through the use of Raeppli, a multicolour lineage tracing tool. The use of Raeppli helps to trace complex tissue movements in a model where temporal and spatial resolution of imaging are limited.
Todd I. Strochlic, Kevin P. Stavrides, Sam V. Thomas, Emmanuelle Nicolas, Alana M. O’Reilly, and Jeffrey R.
Peterson Ack kinase regulates CTP synthase filaments during Drosophila oogenesis EMBO Rep. 15, 1184-1191 (2014).
New muscles, the TARMs (Thoracic Alary-Related Muscles), connect internal organs in the Drosophila embryo.
Body wall muscles attached to the exoskeleton, support larval locomotion. The function of abdominal Alary Muscles (AMs) that dorsally connect to the heart, has remained elusive. We recently discovered new muscles, the Thoracic Alary-Related Muscles (TARMs).
The chosen image is a 3-D reconstructed, dorsal view of the anterior region of a stage 16 embryo. The somatic muscles (ß3-Tubulin, blue) form an outer layer. AMs and TARMs (Org-LacZ, red) attach, at one end to the exoskeleton and at the other end to internal organs : AMs run vertically along segmental borders and connect to the aorta/heart (blue). TARMs display oblique orientation, straddle several segments and connect specific regions of the midgut (dark green). Along their trajectory, AMs and TARMs border specific tracheal branches (bright green). The morphology of AMs and TARMs suggests an architectural role in the internal anatomy of the larva.
Boukhatmi H*, Schaub C*, Bataillé L*, Reim I, Frendo JL, Frasch M, Vincent A.
An Org-1-Tup transcriptional cascade reveals different types of alary muscles connecting internal organs in Drosophila (2014). Development 141, 3761-71. *Contributed equally to this work.
Lasp brings a giant down to size
Lasp, a much shorter version of the actin-binding giant vertebrate protein nebulin, regulates the architecture of Drosophila sarcomeres. Compared to a wild-type myofibril (top), a myofibril from the indirect flight muscle of a fly lacking Lasp (bottom) has shorter, misaligned thin filaments (magenta), and the titin family protein Kettin (green) shows a wider distribution on both sides of the Z-disc. Image taken by structured illumination superresolution microscopy.
Isabelle Fernandes and Frieder Schöck F (2014).
The nebulin repeat protein Lasp regulates I-band architecture and filament spacing in myofibrils. Journal of Cell Biology 206, 559-572
Calcium oscillations in the Drosophila blood-brain barrier relay nutritional information.
Brain development and functions are highly influenced by nutrition. Neural stem cells are able to sense metabolic inputs, and adapt their proliferative behavior accordingly. For example, Drosophila neural stem cells awake from a dormant state upon nutrition, just a few hours after the end of embryogenesis. The blood-brain barrier is a key relay, receiving and converting the nutritional signal into calcium oscillations. Calcium then triggers the release of insulin, that in turn reactivates the neural stem cells.
Movie 1 shows the blood-brain barrier (red) of a young larva that has been feeding for a few hours. The calcium oscillations (green) are strikingly coordinated all along the blood-brain barrier. This allows an efficient release of insulin towards the neural stem cells.
Such critical coordination is achieved via gap junction complexes within the blood-brain barrier. Movie 2 illustrates the loss of coordinated oscillations in a gap junction mutant. Neural stem cells do not reactivate in this mutant.
Gap junction proteins in the blood-brain barrier control nutrient-dependent reactivation of Drosophila neural stem cells.
Spéder P, Brand AH.
Dev Cell. 2014 Aug 11;30(3):309-21.
Dynamic myosin phosphorylation regulates tissue morphogenesis
At the onset of Drosophila gastrulation, prospective mesodermal cells constrict their apices and invaginate into the embryo. The motor protein myosin provides the contractile force for this process, undergoing phases of coalescence and disassembly to constrict cell apices in a step-wise fashion. Prior to ventral tissue invagination, myosin localizes to the basal side of all cells, as myosin re-localizes apically only in ventral cells at the onset of ventral furrow formation. Surprisingly, this re-localization does not requires a change in myosin phosphorylation state. The figure shows cross-sections of embryos stained for myosin and cell membrane expressing wild-type myosin (top row), phospho-mimetic myosin (middle row), or mono-phosphorylatable myosin (bottom row). In all cases myosin is initially basal (left column) and then re-localizes apically (right columns), demonstrating that changes in myosin phosphorylation state are not requires to trigger basal to apical re-localization of myosin.
Vasquez, C.G., Tworoger, M., Martin, A.C.
Dynamic myosin phosphorylation regulates contractile pulses and tissue integrity during epithelial morphogenesis. J Cell Biol. (2014) 206:435-450.
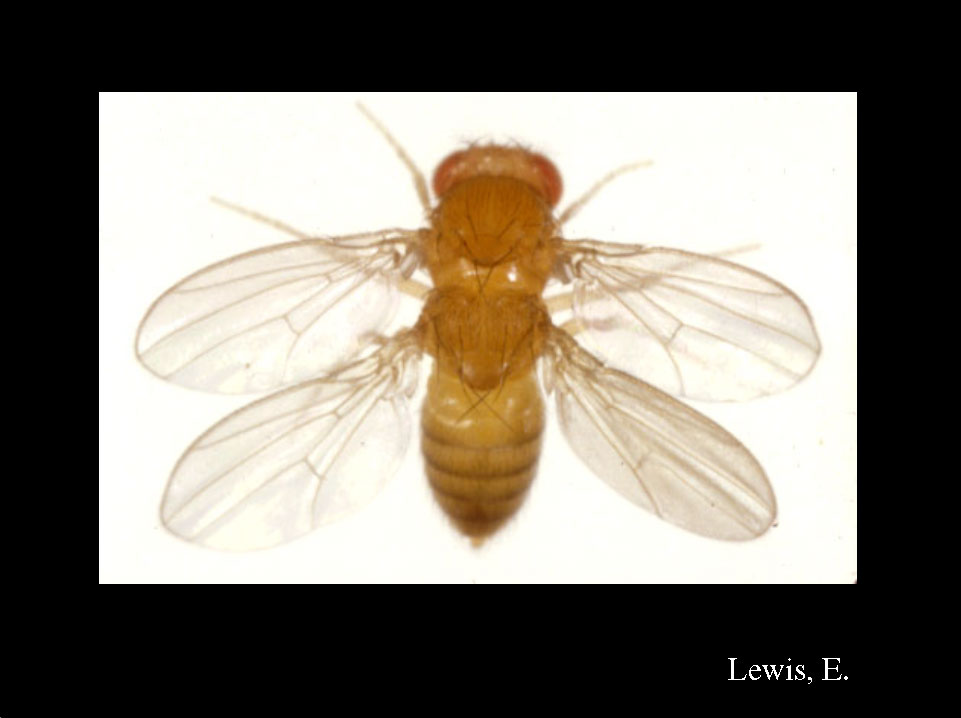