2013 Drosophila Image Award
Winner – Still Image
A complex set of muscles underlying the body wall of third-stage Drosophila larva
The body wall of Drosophila melanogaster larva consists of a chain of metameres divided into anterior (A) and posterior (P) compartments. Hedgehog (Hh) is produced by all the P cells and spreads forwards and backwards into the adjacent A compartments, forming concentration gradients that pattern the A cells. P cells are defined by expression of the transcription factor engrailed (en). The body wall of the larva is underlaid by a complex set of muscles that attach to epidermal tendon cells. These attachments are specified by the expression of stripe and are located in only the A compartments. The image shows a midventral view of three segments of a third-stage Drosophila larva. Note that some muscles span from A cells of one segment to A cells of the next segment; these latter are adjacent to en expressing P cells. Muscles are stained in red with phalloidin; epidermal cells of the posterior compartment (engrailed-expressing cells) are stained in green; nuclei that express stripe are stained in blue. Anterior is towards the top.
Krzemie, J., Fabre, C. C. G., Casal, J., and Lawrence, P. A.
The muscle pattern of the Drosophila abdomen depends on a subdivision of the anterior compartment of each segment.
Development, 139:75–83 (2012).
Winner – Video
Motion input and activation of P1 neurons elicit courtship behaviors
A male fruit fly by its nature courts a female fly. The decision to court depends on integration of multiple sensory inputs such as visual and chemosensory cues from potential mates. Whereas visual information alone is not sufficient to induce courtship behavior in male flies, when a subset of male-specific fruitless and doublesex expressing neurons that respond to chemosensory cues (P1 neurons) were artificially activated, males intensively courted moving objects (even pieces of rubber band as shown in the movie). When stationary, these objects were not courted. These and other results indicate that motion input and activation of P1 neurons are individually necessary and jointly sufficient to elicit courtship behaviors, and provide insights into how courtship decisions are made via sensory integration.
Pan Y, Meissner GW, Baker BS
(2012) Joint control of Drosophila male courtship behavior by motion cues and activation of male-specific P1 neurons.
PNAS 109 (25) 10065-10070; doi:10.1073/pnas.1207107109
Runner-Up
Multiscale imaging of the Drosophila pupa thorax epithelium during metamorphosis.
To understand how individual cell dynamics impact on global tissue morphogenesis we implemented a multiscale imaging method to record the morphogenesis of the Drosophila pupa thorax epithelium during metamorphosis. This was achieved by using multi-position microscopy and reassembling the individual positions after acquisition. This multiscale imaging enabled us to follow ~104 cells over several cell cycles with unprecedented dynamics: 5 min resolution over 26 h of development and 0.32 µm resolution over the ~750×700 µm2 of the tissue. Using cell tracking and image correlation analyses we determined the cell and tissue dynamics and we demonstrated how the Fat/Dachsous/Four-jounted planar cell polarity pathway controls morphogenesis of this epithelium by spatially regulating cell mechanical properties via Dachs Myosin.
The movie features a multiscale imaging of the morphogenesis of the entire Drosophila pupa dorsal thorax epithelium during metamorphosis. Apical cell junctions are labeled with E-cadherin:GFP. The boxed region, highlighting the scutellum, is magnified at the left. The positions of the macrochaetae and of the midline are indicated by white circles and by a black dotted line, respectively. Arrows indicate the anterior-posterior (A/P) and medial-lateral (M/L) axes.
Mechanical control of morphogenesis by Fat/Dachsous/Four-jointed planar cell polarity pathway. 2012.
Bosveld F, Bonnet I, Guirao B, Tlili S, Wang Z, Petitalot A, Marchand R, Bardet PL, Marcq P, Graner F, Bellaïche Y.
Science 336 (6082): 724-727.
Runner-Up
Src controls epithelial tube elongation through polarized cell shape changes
The correct dimensions and shapes of epithelial tubes are crucial for organ function. However, the cellular and molecular mechanisms that mediate initial tube elongation are not well understood. Using genetics and quantitative analyses of single cells, we found a new role of the conserved tyrosine kinase Src42A in epithelial tube morphogenesis. We showed that Src42A acts instructively to induce polarized changes in cell shape and epithelial organization that result in tubular elongation.
The image shows living wild-type (left) and Src42A mutant (right) embryos in which tracheal cells were labelled using the ‘brainbow’ technique. Stochastic expression of different fluorescent membrane markers enabled three-dimensional reconstructions of cell shapes (lower panels). The lumen is shown as a grey tube. By extracting various cell parameters we found that Src42A mutant cells are strongly elongated perpendicular to the tube axis compared to wild-type cells. The longest tube axis is displayed as thin grey lines, while nuclei are shown as blue spheres. These altered cell shapes account for a failure in tube elongation.
Förster D and Luschnig S.
(2012) Src42A-dependent polarized cell shape changes mediate epithelial tube elongation in Drosophila.
Nature Cell Biology 14, 526-534
Asymmetric division of Drosophila male germline stem cell shows asymmetric histone distribution
Using a spatially and temporally controlled gene expression system, we are able to distinguish pre-existing histones from newly synthesized histones. We applied it in the Drosophila male germline stem cell lineage: in the left panel, a transgene expressed canonical histone H3, with pre-existing H3 in green (GFP) and newly synthesized H3 in red (mKO). We compiled images of six mitotic germline stem cells (GSCs) or post-mitotic GSC-gonialblast (GB) pairs, surrounding a representative hub structure (blue). In the right panel, a transgene expressed histone variant H3.3 in a similar manner. We compiled images of six post-mitotic GSC-GB pairs surrounding the hub.
The H3 (left panel) showed an asymmetric segregation pattern with pre-existing H3 (green) preferentially distributed to the GSC side and newly synthesized H3 (red) enriched at the GB side. In contrast, the histone variant H3.3 distributed symmetrically between GSC and GB (orange) in all six GSC-GB pairs. Our findings suggest that stem cells retain pre-existing canonical histones during asymmetric cell divisions in vivo, which may contribute in maintaining their epigenetic memory.
Images generated with data from:
Tran V, Lim C, Xie J, Chen X.
2012. Asymmetric division of Drosophila male germline stem cell shows asymmetric histone distribution. Science. 2012 Nov 2;338(6107):679-82.
(With contributions from Lama Tarayrah and Shandy Lim)
3-D map of the Drosophila genome
Hi-C uncovers multiple scales of chromosome folding in the Drosophila genome. The normalized contact maps for fly embryonic nuclei are shown for the whole genome (left panel), and zoomed-in views of 14 Mb (middle panel) and 2 Mb (right panel) of chromosome 3R. Each element in the map represents the normalized interaction score for a two-dimensional genomic bin. The whole-genome map (left panel) shows that contacts are predominantly constrained within the same chromosome arms, except for the clustering of centromeres and chromosome 4, and the clustering of telomeres. The middle panel zoom shows that the fly genome is organized into discrete physical domains (squares on the diagonal), where contacts are strong within domains but are drastically reduced on crossing domain borders. A significant long-range interaction between the Hox loci BX-C and ANT-C, which are marked by the histone modification H3K27me3, is also depicted. The right panel zoom shows that the physical domains (demarcated by the white lines on the map) are well correlated with various epigenetic mark profiles (a selection is shown above the map), and that the domains can be classified into distinct epigenetic types (three are shown on this map; black “Null” domains, red “Active” domains and blue “Polycomb” domains).
Sexton, Yaffe, Kenigsberg, Bantignies, Leblanc, Hoichman, Parrinello, Tanay and Cavalli.
Three-dimensional folding and functional organization principles of the Drosophila genome.
Cell 148, 458-472 (2012).
Novel Ring Enhancers
Over 6,000 fragments from the genome of Drosophila melanogaster were analyzed for their ability to drive expression of GAL4 reporter genes in the third-instar larval imaginal discs. About 1,200 reporter genes drove expression in the eye, antenna, leg, wing, haltere, or genital imaginal discs. The patterns ranged from large regions to individual cells. The fragments driving similar expression patterns were clustered in different expression groups and their DNA sequence further analyzed for putative candidate binding sites.
In this figure are shown 3 examples of fragments driving expression in ring-like patterns (in green) in the leg (top row) and antenna (2nd row) imaginal discs. The different concentric rings of these two discs are known to outline the different proximo-distal (PD) segments and boundaries of the future corresponding adult appendages. This suggest that these novel “ring enhancers” receive inputs that establish the leg and antenna PD axis.
The bottom panel highlights the significant DNA motifs and putative binding sites found for the enhancer fragments driving a ring-like expression in the leg and/or antenna discs.
Together, all the expression patterns analyzed in this screen have been included in the online FlyLight database and provide a valuable resource to the community as well as they offer a broad overview of how transcriptional regulatory information is distributed in the Drosophila genome.
Aurélie Jory, Carlos Estella, Matt W. Giorgianni, Matthew Slattery, Todd R. Laverty, Gerald M. Rubin, Richard S. Mann
A Survey of 6,300 Genomic Fragments for cis-Regulatory Activity in the Imaginal Discs of Drosophila melanogaster
Cell Reports. 2012 Oct 25;2(4):1014-24
Blocking Shaker K+ channels rescues a Drosophila model of SMA – Brian D. McCabe, Columbia University
Spinal Muscular Atrophy (SMA) is a motor system disease caused by mutations in the evolutionarily conserved survival motor neuron (SMN) gene. SMA is the most common inherited cause of infant mortality and currently there is no treatment. Using a Drosophila model of SMA we found that, contrary to previous hypotheses, the disease was not caused by autonomous defects in motor neurons or muscles, but originated from a disruption of motor circuit function, which subsequently perturbed motor neurons and muscles. This was a important first demonstration that a neurodegenerative disease could be induced by the dysfunction of neuronal circuits. Furthermore, genetic inhibition of K+ channels or administration of the FDA approved small molecule K+ channel blocker 4-AP restored motor circuit activity and provided benefit in the Drosophila SMA model. Based on this data 4-AP is now in human clinical trials for SMA at both Columbia University and the NIH, in a pioneering example of a drug moving directly from a Drosophila disease model to human clinical trials.
Image: 10 superimposed 60 second larval locomotion traces from control, smn mutants and smn mutants expressing Shaker Dominant Negative (UAS-SDN) in cholinergic neurons (Cha-Gal4). Normal locomotion is fully restored by the inhibition of the K+ channels in cholinergic motor circuit neurons.
Reference: Imlach, W.L., Beck, E.S., Choi, B.J., Lotti, F., Pellizzoni, L., and McCabe, B.D.
(2012). SMN Is Required for Sensory-Motor Circuit Function in Drosophila.
Cell 151, 427-439
The peripheral nervous system as hematopoietic microenvironment
Recent studies have demonstrated that hematopoiesis in the Drosophila larva depends on the peripheral nervous system (PNS). Regulation of stem cell niches and tissue microenvironments by anatomical and molecular connections of the PNS is a common theme that is being uncovered across species.
Fillet preparation of a Drosophila larva showing hemocytes (red) in close contact with peripheral neurons (green). Anterior left, dorsal center.
Makhijani K and Brückner K.
(2012) Of blood cells and the nervous system: hematopoiesis in the Drosophila larva.
Fly 6(4).
Drosophila female reproductive gland formation
The spermathecal and parovian glands, each connected to the Drosophila female reproductive tract with a long tubular duct, were visualized with RFP reporter (red) marking Lozenge-expressing cells and GFP reporter (green) marking the descendants of Lozenge-expressing cells during development. The smaller cells with both GFP and RFP expression are epithelial cells, while larger cells with GFP expression are secretory cells. Using the clonal analysis, we found that each secretory cell arises from a three-cell secretory unit derived from a Lozenge+ precursor in female genital disc during pupal development. All nuclei are stained with DAPI (blue).
Sun, J. and Spradling, A. C. (2012).
NR5A Nuclear Receptor Hr39 Controls Three-Cell Secretory Unit Formation in Drosophila Female Reproductive Glands.
Curr Biol 22, 862-871.
Laser surgery reveals adaptive function of microscopic morphology
Investigating the functional morphology of microscopic structures in Drosophila via manipulative experimentation has previously been restricted to genetic engineering and/or the use of mutant stocks, both of which cannot easily exclude the potentially confounding effects of pleiotropy. Phenotypic engineering avoids such caveats, but has typically been impractical, imprecise, or impossible to perform at a microscopic level. This image demonstrates the precision of a micron-scale laser surgery technique used here to study the adaptive function of male genital spines in Drosophila ananassae (near spine partially ablated, far spine intact for reference). Note the absence of collateral damage to surrounding structures and bristles. Though applications for this technique are seemingly limitless, this study tested the hypothesis that the genital spines of D. ananassae function prior to mating to promote competitive male copulation success. Incremental reductions in male genital spine length progressively reduced male copulation success, males completely lacking genital spines could not achieve copulation, and males with partially ablated versus intact genital spines tended to lose the competition for access to a single virgin female, despite similar rates of copulation attempts and female resistance behaviors.
Grieshop, K. and Polak, M. (2012).
The pre-copulatory function of male genital spines in Drosophila ananassae [Doleschall] (Diptera: Drosophilidae) revealed by laser surgery.
Evolution. 66:2637-2645. DOI: 10.1111/j.1558-5646.2012.01638.x
Picture credit: Necati Kaval, University of Cincinnati
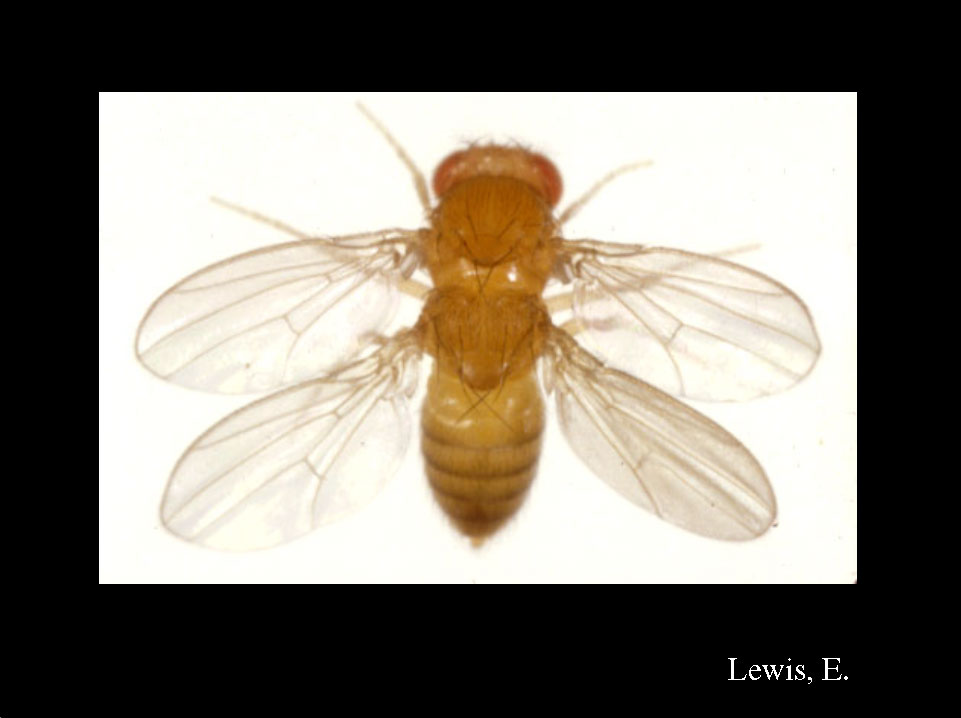