2011 Drosophila Image Award
Fluorescent sperm in the female sperm-storage organ of Drosophila melanogaster
Sperm competition occurs after females mate with multiple males whose sperm compete for fertilizations within the female reproductive tract. Our understanding of events during sperm competition has been constrained by the challenge of directly observing sperm behavior and fate inside the female and the inability to differentiate sperm from multiple males. This image shows the primary sperm-storage organ (seminal receptacle) of a female Drosophila melanogaster that has mated to transgenic males with green (first male) or red (second male) fluorescently-labeled sperm heads. Sperm competition mechanisms elucidated by these novel transgenics include active sperm motility, sperm displacement and female ejection.
Mollie K. Manier, John M. Belote, Kirstin S. Berben, David Novikov, Will T. Stuart, and Scott Pitnick.
Resolving mechanisms of competitive fertilization success in Drosophila melanogaster.
Science 328, 354-357 (2010).
Genetic dissection and visualization of the Drosophila courtship neuronal network
The approximately 1500 fruitless expressing neurons in the Drosophila nervous system orchestrate the innate and complex male courtship ritual. We used genetic methods to define 100 morphologically distinct neuronal groups, which we believe represent functional units within this complex network. We visualized different neuronal groups (left panel) by expressing GFP (green) and used image registration to constructed a 3D model of the nervous system (brain shown in grey) and digitize the fruitless neurons (colored traces). This data allowed us to construct a map of how different neuronal groups could be connected (right panel). The map gave us clues as to how the different components in the network can transform sensory information to instruct motor centers to generate the intricate behavior sequences during courtship. Our genetic approach gave us the necessary tools to test our hypotheses and advance towards a cellular understanding of the sensory to motor transformation that takes place in the nervous system during behavior.
Yu,J.Y., Kanai,M.I., Demir,E., Jefferis,G.S., and Dickson,B.J. (2010).
Cellular organization of the neural circuit that drives Drosophila courtship behavior.
Curr. Biol. 20, 1602-1614.
Ovarian Organogenesis
To understand how organs form, it is necessary to understand not only how each cell type is specified but also how cells cooperate to form appropriate organ shapes and tissue architectures. We used live imaging of developing egg chambers to show that the basal surfaces of follicle cells undergo a series of directional, oscillating contractions driven by periodic myosin accumulation on a polarized actin network. Inhibition of the actomyosin contractions or their coupling to extracellular matrix (ECM) blocked tissue elongation, whereas enhancement of the contractions exaggerated it. Our findings reveal a novel mechanism controlling organ shape and a new experimental model for the study of the regulation of oscillatory actomyosin activity.
Tissue elongation requires oscillating contractions of a basal actomyosin network.
He L, Wang X, Tang HL, Montell DJ.
Nat Cell Biol. 2010 Dec;12(12):1133-42. Epub 2010 Nov 21.
Picture credit: Ho Lam Tang
Light-avoidance-mediating photoreceptors tile the Drosophila larval body wall
The body wall of the Drosophila larva is covered by the dendrites of a regular array of photosensing neurons, which allow the larva to detect and avoid harmful light. This image shows the dorsal view of a Drosophila larva with the light avoidance-mediating photoreceptors labeled in green and the posterior epidermal cells of each segment labeled in red.
Xiang Y, Yuan Q, Vogt N, Looger LL, Jan LY, Jan YN.
Light-avoidance-mediating photoreceptors tile the Drosophila larval body wall.
Nature 468, 921-6 (2010)
Picture credit: Chun Han, UCSF.
A Drosophila testis is shown with elongating spermatids during the vital apoptosis-like process called “individualization”. During this process, an actin-based complex (called the individualization complex [IC] and is shown in gold in the middle inset) translocates from nuclear heads (top, blue; also in the upper inset) to tail ends of spermatids (bottom; also in the lower inset). This caudal movement of the IC drives the removal of the spermatids’ bulk cytoplasmic contents into a membranal sack called “cystic bulge” (red bulges; also in the middle inset), which eventually is pinched off as a “waste bag” (large red ovals in the bottom). Soti (green), an inhibitor of a ubiquitin ligase complex required for caspase activation in this process, is expressed in a gradient from tail ends (bottom) to nuclear heads (top, blue) of the spermatids. Consequently, caspase activation (activated effector caspase, red) occurs in an inverse graded fashion, such that the regions of the developing spermatid that are the last to individualize experience the lowest levels of activated caspases. This unique spatial regulation of caspase activation assures caspase-dependent differentiation while preventing full-blown apoptosis.
Yosef Kaplan, Liron Gibbs-Bar, Yossi Kalifa, Yael Feinstein-Rotkopf and Eli Arama.
Gradients of a ubiquitin E3 ligase inhibitor and a caspase inhibitor determine differentiation or death in spermatids.
Dev Cell, 19, 160-173 (2010).
Microtubules treadmill throughout the cytoplasm in cells depleted of Patronin
Microtubules in Drosophila S2 cells are arranged with stable minus ends in the cell body and dynamic plus ends near the cell periphery (left). While much is known about the regulation of plus end dynamics, the protein(s) responsible for regulating the stability of the minus end remain unknown. We have identified a new microtubule binding protein, named Patronin, that stabilizes the microtubule minus ends. When cells expressing GFP-tubulin are depleted of Patronin (right), microtubule minus ends undergo constant depolymerization, resulting in short microtubules that treadmill throughout the cell.
Goodwin, S.S., and Vale R.D.
Patronin regulates the microtubule network by protecting microtubule minus ends.
Cell, 143, 263-74 (2010).
Genetic Magic Trick – Refining Expression by Intersection
The Drosophila toolbox has been greatly expanded with the introduction of the Q repressible binary expression system: the Q system. Analogous to the GAL4 system, the Q system contains the transcription factor QF, the QF suppressor QS, and the QF reporter QUAS-geneX. The new system allows for GAL4-independent labeling and manipulation of tissues, mosaic analysis, and unprecedented control over the refinement of expression patterns.
An enhancer trap often expresses in more tissues than desired. The ability to refine such an expression pattern would be advantageous. On the left is an adult brain showing the expression pattern from the NP21-GAL4 enhancer trap. On the right is a brain showing the GH146-QF expression pattern. These two expression patterns can be used together to limit expression only to where both lines are expressed- the “AND” intersectional. As a result, only a very small subset of neurons are labeled (bottom). Neuropil are labeled by staining with the nc82 antibody (purple).
Genotypes: left, NP21-GAL4, UAS-mCD9GFP; right, GH146-QF, QUAS-mCD8GFP; bottom, NP21-GAL4, GH146-QF, QUAS-FLP, UAS>stop>mCD8GFP. ‘>’, FRT.
Potter CJ, Tasic B, Russler EV, Liang L, Luo L.
(2010) The Q System: A Repressible Binary Expression System for Transgene Expression, Lineage Tracing and Mosaic Analysis.
Cell, 141:536-548.
Secretory activity acts locally to drive tracheal tube expansion
Tracheal tube expansion in Drosophila involves apical secretion and deposition of a luminal matrix, but the role of secretion and the nature of forces involved in the process were not clear. We used mutations in the Sec24 homolog stenosis (sten), which cause a failure in luminal transport of secreted proteins, such as Vermiform (Verm; magenta) and a defect in dilation of the tracheal lumen (labeled with Chitin; green). We used Abdominal-B (Abd-B)-Gal4 to restore secretion in sten mutants in the posteriormost tracheal metamere. Note that tube dilation and cuticle formation, as well as Verm secretion, are rescued exclusively in the Abd-B-Gal4-expressing posterior part of the tube, while the anterior part remains narrow. These results suggest that local secretion-dependent events, rather than extrinsic cues (such as luminal pressure), drive the tube dilation process.
Förster D, Armbruster K, Luschnig S.
(2010) Sec24-dependent secretion drives cell-autonomous expansion of tracheal tubes in Drosophila.
Current Biology 20, 62-68
H2O2 May Be the Universal Immediate Damage “Wound Signal”
We wanted to identify the signal released by cell damage responsible for recruiting Drosophila macrophages (hemocytes) to wound sites. Therefore, we tested a number of likely candidates by using a combination of genetic and pharmacological approaches utilizing a heparin bead grafted into the ventral epithelium in a way that both generates a wound and enables delivery of drug to the wound site (as illustrated in the figure). The confocal images show typical hemocyte recruitment to control beads (upper left panel) or those soaked in apyrase (top right panel), ATP--S (bottom left panel) and diphenyleneiodonium (DPI) (bottom right panel), 1 hr after implantation. A previous study in zebrafish larvae reported that H2O2 originating from the wound epithelium was responsible for attracting neutrophils to a wound. Knockdown of this gradient with DPI, which inactivates the NADPH oxidases responsible for generating H2O2, blocked the wound inflammatory response. To examine whether such a rapid wound signal might be conserved across phyla, we loaded beads with DPI and saw a dramatic block of all hemocyte migration to the bead, suggesting that a ROS, possibly H2O2, is indeed responsible for drawing hemocytes to wounds in the Drosophila embryo as well. These results were further corroborated by RNAi knockdown of Duox, the enzyme responsible for generation of H2O2, specifically in the embryonic epidermis. RNAi knockdown reduces recruitment of hemocytes to less than 50% of control wounds. Taken together, these data suggest that the primary cue directing hemocytes to embryonic wounds is H2O2.
Moreira S, Stramer B, Evans I, Wood W, Martin P.
“Prioritization of competing damage and developmental signals by migrating macrophages in the Drosophila embryo.”
Curr Biol. 2010. 20(5): 464-470.
Identification of a new actin regulatory protein: Mical remodels actin filaments and induces bristle branching
How instructive cues present on the cell surface exert their precise effects on the actin cytoskeleton is poorly understood. We now identify a new actin filament disassembly factor Mical that provides a direct physical and functional link between Semaphorins, one of the largest families of extracellular guidance cues, and the modification of the actin cytoskeleton. In these examples, normally unbranched, actin-rich bristle cell processes (macrochaetae) undergo dramatically branched morphological changes in response to increased Mical expression (photomicrographs of adult bristles). In the bottom two images, branching is so extensive that adjacent bristles have intertwined with one another and appear to be fused together.
Ruei-Jiun Hung, Umar Yazdani, Jimok Yoon, Heng Wu, Taehong Yang, Nidhi Gupta, Zhiyu Huang, Willem J.H. van Berkel, and Jonathan R. Terman
(2010) Mical links semaphorins to F-actin disassembly
Nature, 463: 823-827.
In toto 4d recording of Drosophila embryogenesis captured using Selective Plane Illumination Microscopy (SPIM). The multi-view SPIM acquisition was registered using a sample independent bead-based registration framework. Three arbitrary views of the same specimen are rendered in 3d with POV-ray.
Stephan Preibisch, Stephan Saalfeld, Torsten Rohlfing and Pavel Tomancak
(2010) Software for bead-based registration of selective plane illumination microscopy data
Nat. Methods 7(6), 418-419
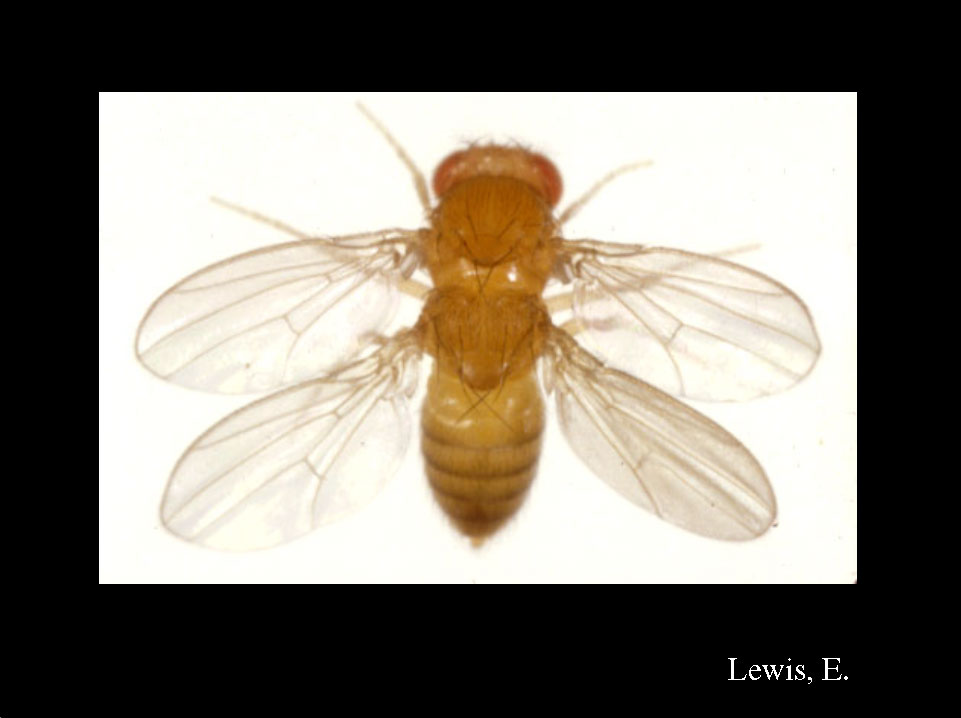